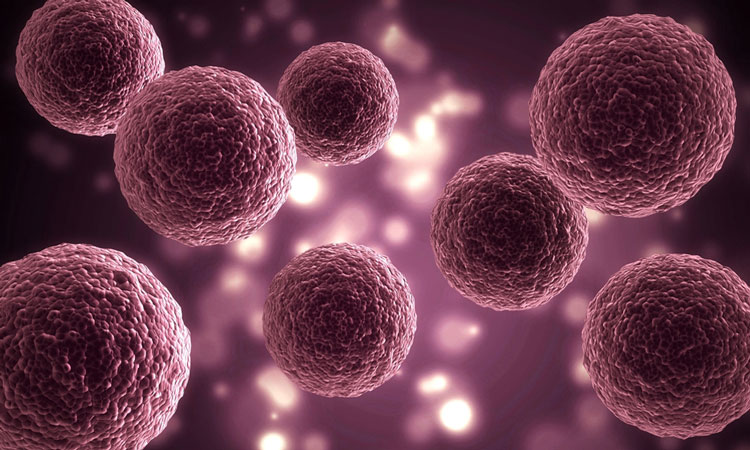
Imagine being able to create an in vitro replica of a diseased organ to study the molecular mechanism underlying the illness. Now take a step further: envision testing drugs in these organoids to identify the ones that can treat disease safely and effectively without needing to run expensive clinical trials first. Further still, think about implanting these mini organs into the patient to restore lost function. With multiple breakthroughs in recent decades, these goals are now much closer to reality.
Creating replicas of organs in vitro has been a goal of researchers for over a century. Early efforts include the pioneering work of Ross Harrison, who studied the growth of living nerve fibres from frog embryos in a hanging droplet tissue culture system.1 This early work laid the foundation for a century of research on finding the optimal conditions for growing parts of organs in in vitro cultures, testing their functions and even mimicking many diseases.
Pioneering 3D cell culture for normal and cancer cells
A key breakthrough in the development of organoid cultures came with the establishment and demonstration that when normal breast epithelial cultures were placed in a gel-like reconstituted basement membrane, they formed organised fluid-filled spherical structures, essentially recapitulating the tubular or acinar structures of normal mammary glands in vitro. Notably, when a series of breast cancer cells were subjected to the same three-dimensional matrix, none formed the organised spheroids, instead forming disorganised multicellular structures reminiscent of various stages of breast cancer.2-4 This series of landmark studies formed the basis for subsequent breakthroughs in breast cancer research that would not have been observable in normal two-dimensional cell cultures.
Replicating complex human biology in vitro
Organs in vivo are made up of several different cell types in close proximity that cooperate with one another. In light of the natural composition of organs, a formal definition of an ‘organoid’ might be a mini organ in a dish that comprises all or most of the various cell types found in the organ being modelled. Efforts to create such organoids with multiple cell types was boosted by two breakthrough discoveries published within six months of each other. The first was the finding that embryonic stem cells (ESC) under specific 3D culture conditions, can grow into brain-like organoids with specific regions that mimic the development of the different layers of the cerebral cortex.5,6 For the first time, the complex steps of brain development could be observed live and studied in vitro. Shortly thereafter, scientists demonstrated that single adult intestinal stem cells can form the complex architecture of intestinal crypts and villi, containing all four differentiated cell-types that make up the intestine.7 This second breakthrough demonstrated that intestinal stem cells can form self-organising 3D organoids on their own without support from non-intestinal cells. Curiously, these organoids can be maintained in culture and studied for years.
Organoids have been developed for the pancreas, stomach, colon, liver, kidney, thyroid, and heart, among others.8 With the widespread use of induced pluripotent stem cells (iPSCs), organoids can now be generated from the skin or blood cells of both healthy volunteers and disease patients, opening the door to studying various diseases and creating individually customised potential treatments.
Modelling and studying complex interactions
Imagine being able to study the complex interactions between different cell types in the brain that are required for normal brain function, and the dysfunctions that occur in a complex disease like Alzheimer’s or schizophrenia.9,10 To reach this goal, the next generation of organoid development approaches use combinations of different cell types together in a 3D culture to form a more complex organoid that better mimics the complexity of tissues. One remarkable example of these ensembles is gut organ cells that were co-cultured with neural crest cells that together matured into gut organoids containing a nervous system that produced wave-like contractions of the gut tissue. 11 These organoid assemblies or ‘assembloids’ have been used to successfully model complex neural circuits, brain development, neurological disorders and brain cancer. 12
Testing drugs in vitro in organoid cultures
A clear validation of the utility of organoids is in drug discovery and development. Patient-derived tumour tissue samples are grown in organoid cultures. These better represent the in vivo tumour microenvironment since they contain tumour stromal tissue, blood vessels, and so on. Now It is possible to test drugs in tumour organoids derived from individual patients’ biopsies to better understand their drug sensitivity and resistance profile and then tailor treatment accordingly. A large body of evidence demonstrates that these platforms can accurately predict tumour response to drugs. In multiple studies, cancer drugs tested on patient-derived organoids correctly predicted treatment outcomes with greater than 80 percent accuracy, demonstrating the clear utility of these organoids in outcomes prediction and optimisation of dose and regimen.13-18
Confidence in organoid-generated data that translates in vitro efficacy to efficacy in the clinic is growing. Recently, the US Food and Drug Administration allowed the existing drug sutimlimab to enter clinical trials for a new disease indication based on efficacy data generated in organoid cultures. A logical extension of this approach is in the discovery of new drugs, and many companies are utilising tumour organoids in their drug-discovery programs. As we continue to evolve our drug characterisation processes at Verseon, we see the extensive use of assembloids as essential enabling technology for the future of all drug development.
Organoids for implantation
With the advent of iPSCs as source material, an exciting possibility is growing organoids for implantation back into the donor for organ repair. Autologous tissue transplantation holds the promise of repairing damaged organs without the need for donor organs or the risk of immune rejection.
Kidney repair is one area of urgent need. Kidneys perform essential functions such as managing blood filtration, the balance of water and electrolytes, blood pressure, and hormone production. Once damaged, kidneys do not regenerate or repair on their own. Approximately 13 percent of the world’s population is affected by kidney disease and for most of them the only two options are dialysis or transplantation of donor kidneys.19 A promising area of ongoing research has been the development of functional kidney organoids for implantation.
Although much progress still needs to be made before we are ready to regenerate kidney function using organoids, some significant breakthroughs have occurred. It is now possible to make human stem cell-derived kidney organoids that contain kidney nephrons, collecting ducts and capillary endothelial cells, which make up the basic unit of filtration inside a kidney.20 These have now been implanted into mouse kidneys and were found to attract host blood vessels into the organoids, mimicking the glomerular filtration barrier.21 This result is a tantalising sign that kidney organoid implantation can lead to incorporation and maturation of the implanted tissue into functional units. Although much work still lies ahead, success in this area could alleviate the suffering of millions of kidney disease patients worldwide.
Restoring and enhancing organ function
As research on the development of organoids becomes increasingly sophisticated, it leads to the exciting prospect that one day each of us could have an in vitro organoid ‘twin’ for the purpose of diagnosing illnesses, tailoring treatments, and use in tissue regeneration. It is already possible to observe organ dysfunction by either collecting patient stem cell samples or inducing pluripotent stem cells, developing organoids, and generating multi-omics data to diagnose illnesses. Tailoring treatments for each patient’s needs is the next logical step, where treatment outcomes can be predicted accurately based on the organoid response. Cancer treatment is the most active area in which organoid ‘twins’ are being used to find the most effective personalised treatments.
The next frontier is regeneration of organs to compensate for compromised function. For functional compensation, the implantation of organoids is proving to be a promising option. Aside from kidney repair, some other areas of active research include the transplantation of organoids to repair retinal degeneration and to repair colon injury.22-26 Technically, these findings could apply to both restoration and enhancement of organ function.
Organoids will play increasingly crucial roles not only in research and diagnostics, but also in therapies to restore and enhance the human body. Continuing research on organoids will expand the number of human organs being replicated as organoids in vitro and will represent each complex tissue with greater fidelity. As this trend continues, we can look forward to a future where organoid implantation for organ repair and enhancement becomes a part of standard medical care.
References
- Harrison RG. Observations on the living developing nerve fiber. Exp Biol Med. 1906;4:140-3.
- Barcellos-Hoff MH, Aggeler J, Ram TG, Bissell MJ. Functional differentiation and alveolar morphogenesis of primary mammary cultures on reconstituted basement membrane. Development. 1989;105(2):223-35.
- Petersen OW, Ronnov-Jessen L, Howlett AR, Bissell MJ. Interaction with basement membrane serves to rapidly distinguish growth and differentiation pattern of normal and malignant human breast epithelial cells. Proc Natl Acad Sci U S A. 1992;89(19):9064-8.
- Simian M, Bissell MJ. Organoids: A historical perspective of thinking in three dimensions. J Cell Biol. 2017;216(1):31-40.
- Eiraku M, Watanabe K, Matsuo-Takasaki M, Kawada M, Yonemura S, Matsumura M, et al. Self-organized formation of polarized cortical tissues from ESCs and its active manipulation by extrinsic signals. Cell Stem Cell. 2008;3(5):519-32.
- Lancaster MA, Renner M, Martin CA, Wenzel D, Bicknell LS, Hurles ME, et al. Cerebral organoids model human brain development and microcephaly. Nature. 2013;501(7467):373-9.
- Sato T, Vries RG, Snippert HJ, van de Wetering M, Barker N, Stange DE, et al. Single Lgr5 stem cells build crypt-villus structures in vitro without a mesenchymal niche. Nature. 2009;459(7244):262-5.
- Yang S, Hu H, Kung H, Zou R, Dai Y, Hu Y, et al. Organoids: The current status and biomedical applications. MedComm (2020). 2023;4(3):e274.
- Lin YT, Seo J, Gao F, Feldman HM, Wen HL, Penney J, et al. APOE4 Causes Widespread Molecular and Cellular Alterations Associated with Alzheimer’s Disease Phenotypes in Human iPSC-Derived Brain Cell Types. Neuron. 2018;98(6):1294.
- Sekar A, Bialas AR, de Rivera H, Davis A, Hammond TR, Kamitaki N, et al. Schizophrenia risk from complex variation of complement component 4. Nature. 2016;530(7589):177-83.
- Workman MJ, Mahe MM, Trisno S, Poling HM, Watson CL, Sundaram N, et al. Engineered human pluripotent-stem-cell-derived intestinal tissues with a functional enteric nervous system. Nat Med. 2017;23(1):49-59.
- Pasca SP. Assembling human brain organoids. Science. 2019;363(6423):126-7.
- Bleijs M, van de Wetering M, Clevers H, Drost J. Xenograft and organoid model systems in cancer research. EMBO J. 2019;38(15):e101654.
- Drost J, Clevers H. Organoids in cancer research. Nat Rev Cancer. 2018;18(7):407-18.
- Vlachogiannis G, Hedayat S, Vatsiou A, Jamin Y, Fernandez-Mateos J, Khan K, et al. Patient-derived organoids model treatment response of metastatic gastrointestinal cancers. Science. 2018;359(6378):920-6.
- Ooft SN, Weeber F, Dijkstra KK, McLean CM, Kaing S, van Werkhoven E, et al. Patient-derived organoids can predict response to chemotherapy in metastatic colorectal cancer patients. Sci Transl Med. 2019;11(513).
- Yao Y, Xu X, Yang L, Zhu J, Wan J, Shen L, et al. Patient-Derived Organoids Predict Chemoradiation Responses of Locally Advanced Rectal Cancer. Cell Stem Cell. 2020;26(1):17-26 e6.
- Lago C, Federico A, Leva G, Mack NL, Schwalm B, Ballabio C, et al. Patient- and xenograft-derived organoids recapitulate pediatric brain tumor features and patient treatments. EMBO Mol Med. 2023;15(12):e18199.
- Kalejaiye TD, Barreto AD, Musah S. Translating Organoids into Artificial Kidneys. Curr Transplant Rep. 2022;9(4):276-86.
- Takasato M, Er PX, Chiu HS, Maier B, Baillie GJ, Ferguson C, et al. Kidney organoids from human iPS cells contain multiple lineages and model human nephrogenesis. Nature. 2015;526(7574):564-8.
- van den Berg CW, Ritsma L, Avramut MC, Wiersma LE, van den Berg BM, Leuning DG, et al. Renal Subcapsular Transplantation of PSC-Derived Kidney Organoids Induces Neo-vasculogenesis and Significant Glomerular and Tubular Maturation In Vivo. Stem Cell Reports. 2018;10(3):751-65.
- Shirai H, Mandai M, Matsushita K, Kuwahara A, Yonemura S, Nakano T, et al. Transplantation of human embryonic stem cell-derived retinal tissue in two primate models of retinal degeneration. Proc Natl Acad Sci U S A. 2016;113(1):E81-90.
- Assawachananont J, Mandai M, Okamoto S, Yamada C, Eiraku M, Yonemura S, et al. Transplantation of embryonic and induced pluripotent stem cell-derived 3D retinal sheets into retinal degenerative mice. Stem Cell Reports. 2014;2(5):662-74.
- Mandai M, Fujii M, Hashiguchi T, Sunagawa GA, Ito SI, Sun J, et al. iPSC-Derived Retina Transplants Improve Vision in rd1 End-Stage Retinal-Degeneration Mice. Stem Cell Reports. 2017;8(1):69-83.
- Yui S, Nakamura T, Sato T, Nemoto Y, Mizutani T, Zheng X, et al. Functional engraftment of colon epithelium expanded in vitro from a single adult Lgr5(+) stem cell. Nat Med. 2012;18(4):618-23.
- Fordham RP, Yui S, Hannan NR, Soendergaard C, Madgwick A, Schweiger PJ, et al. Transplantation of expanded fetal intestinal progenitors contributes to colon regeneration after injury. Cell Stem Cell. 2013;13(6):734-44.
About the author
Anirban Datta, Head of Discovery Biology at Verseon International Corporation
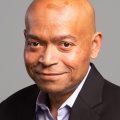
Anirban Datta PhD is the head of discovery biology at Verseon International Corporation. He has over 20 years’ experience in biomedical research and pharmaceutical drug discovery. He is the driving force behind Verseon’s automated processes for biological characterisation of compounds, teasing out their unique properties, and structuring drug candidate development pathways. He has led multiple drug discovery programs in diverse disease areas, including cardiometabolic disorders, ophthalmology, and oncology. Datta was previously a scientist and Susan B. Komen Breast Cancer Foundation Fellow at UCSF and the recipient of lung and breast cancer concept awards from the US Department of Defense. His early research was spun out into a cancer diagnostics company. He received his BS in physics and biology from the University of Chicago and his PhD in molecular biology from the University of Pennsylvania.